New model for reproduction of E. coli bacteria
With a new model, AMOLF researchers reveal how single celled organisms like bacteria coordinate growth, cell division and DNA replication. Bacteria reproduce via growth and cell division. During each cycle of growth and division, the so-called cell cycle, the cell needs to copy all cellular components exactly once. How the cell achieves this has been a long-standing question in the field. PhD student Mareike Berger and professor Pieter Rein ten Wolde have now developed a mathematical model that offers an explanation. They publish their findings on November 7 in Nature Communications.
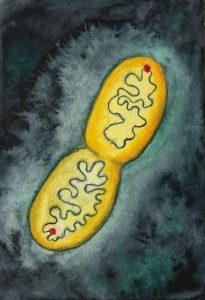
The question of how a bacterium ‘knows’ when it is time to copy its DNA, has occupied scientists for decades. “The first quantitative experiments with the model organism E. coli date back to the 1950s and we still don’t know exactly how these bacteria ensure that they multiply stably”, says Berger. Recent developments in single-cell microscopy allow researchers to look at individual cells and observe them while they are growing and dividing. It seems that E. coli follows some basic rules, Berger explains: “The bacterium grows by continuously increasing its volume. When the bacterium reaches a well-defined volume, it starts a new round of DNA replication. This simple rule ensures that cells make precisely one copy of the chromosome during each division cycle. But what we still don’t understand is how the cell measures its own volume, such that it knows when to start a new round of DNA replication.”
Initiator protein
A new round of replication always starts at one specific site on the chromosome, the so-called origin of replication. It is clear from biological experiments that opening the chromosome requires an activator protein (DnaA) that binds to the origin. This protein can, however, also strongly bind to other sites on the chromosome.
Berger says: “In the 1990s, this information was used to devise the so-called ‘titration model’, in which DnaA first binds to these titration sites, and only when all of these sites have been saturated, does it bind to the origin to start a new round of replication. We tested this titration model under different growth conditions and found to our surprise that it only produces stable cell cycles for low growth rates. E. coli can, however, grow even faster than the time it takes to replicate the entire chromosome. At such fast growth rates, there must be another mechanism with which the bacteria keep their cell cycles stable.”
Protein activation switch
Many experiments have shown that the activator protein DnaA can take an active and an inactive form and that it switches from one state to the other over the course of the cell cycle via several mechanisms. Berger, therefore, developed a new mathematical model for the regulation of replication initiation, which takes this protein activation switch into account. “We found that a model based on this protein activation switch exhibits stable cell cycles at low and high growth rates”, she says.
However, the switch model overlooks the biological observation that DnaA binds to titration sites on the chromosome before it becomes available for binding the origin. “We therefore combined the switch model with the titration model, and to our surprise the combined model is more robust than the models based on either mechanism alone. Even when we introduced noise – which is always present in biological systems – the cell cycles remained stable, at both low and high growth rates,” Berger says. “Our modelling therefore predicts that DNA replication is controlled by both titration and activation. By turning parts in our model on or off, these predictions can now be tested with biological experiments, so that we can ultimately discover how bacteria regulate their cell cycle.”
Synthetic cell
Berger’s research is part of the NWO program BaSyC (Building a Synthetic Cell), which aims to make an autonomous, self-reproducing cell from non-living molecular building blocks. Researchers from five Dutch universities and various AMOLF groups work in the program. They have backgrounds in physics, chemistry, biology and even philosophy. Berger: “By creating a working cell with a bottom-up approach, we hope to not only better understand how life works, but also to find solutions for when it does not work. For example, better knowledge about how bacteria grow and divide can be used to develop smarter antibiotics. With our own research on cell cycle models, we hope to generate insights about how cells make robust copies of themselves in nature, that can eventually be implemented in an artificial cell.”
Reference
Mareike Berger and Pieter Rein ten Wolde, Robust replication initiation from coupled homeostatic mechanisms, Nature communications, 7 November (2022).
doi:10.1038/s41467-022-33886-6